Transportation
Light-Duty Vehicles
Model Overview
The light-duty vehicles model in US-REGEN characterizes the fleet composition and energy use associated with light-duty vehicles for personal transportation. Like the buildings model, the passenger transport model begins by using detailed data to allocate service demand by technology within modeled household structural classes. Data for vehicle ownership by building type and settlement type come from the American Community Survey (ACS), a part of the US Census. Data for vehicle types, age, and utilization come primarily from the 2017 National Household Travel Survey (NHTS), which is a U.S. Department of Transportation Federal Highway Administration dataset that provides comprehensive historical information on travel and transportation patterns in the United States.
The model captures granularity in the vehicle and household population along several relevant dimensions. In each model region, personal light-duty vehicles are characterized by:
Household characteristics
- Building type (detached single-family vs. multi-family / other)
- Settlement type (six classes ranging from high-density urban to rural)
- Access to charging (home only, work only, home and work, and neither, i.e. public only)
- Number of vehicles owned (one, two, or three and greater)
- Vehicle order (primary, secondary, tertiary)
- Driving intensity (high, medium, and low vehicle miles traveled per vehicle)
Vehicle characteristics
- Vehicle class (car, SUV, pickup truck, and van)
- Vehicle age
The model projects total service demand by time and model region based on household growth rates (which are constructed from the same population projections as the building model). Exogenous assumptions about the turnover of the existing fleet are based on vintage estimates from the NHTS dataset and assumed survival rates of vehicles. Scrappage lifetimes for vehicles come from the Bandivadekar, et al. (2008), "On the Road in 2035" study, with the option for endogenous early retirement, i.e. accelerated scrappage. The model tracks turnover and purchase decisions for each vehicle in each household type, accounting for the shift from primary to secondary and tertiary (i.e. less driven) as vehicles age.
The decision model assesses the total cost for discrete technological options in each vintage across the explicit structural classes. Vehicle technology types include internal combustion engine vehicles (ICEVs), battery electric vehicles (BEVs) with varying range, plug-in hybrid electric vehicles (PHEVs), hydrogen fuel cell vehicles (HFCVs) and a mobility-as-a-service option. The model constructs a total cost of ownership metric for each vehicle in each structural class including upfront capital costs (including charging infrastructure for electric vehicles) and annual operating costs (including delivered fuel costs, maintenance, fixed operating costs such as parking and insurance, and disutility costs associated with range and refueling). While vehicle capital costs are assumed to be identical across regions and household types, annual operating costs vary across structural classes.
US-REGEN characterizes trade-offs among vehicle options from the consumer perspective using a nested logit model, which determines market shares as a function of relative total cost of ownership. The discrete options and logit nesting structure are shown in Figure 1. The parameters in each nest indicate the magnitude of the random variation term reflecting unobserved costs in the logit formulation, in thousands of dollars (net present value). As this parameter approaches zero, the decision between elements of the nest approaches a pure optimization, that is, 100% of market share is achieved by the option with the lowest (observed) cost. Larger parameter values indicate greater heterogeneity of unobserved costs and thus more variation in market shares across options.
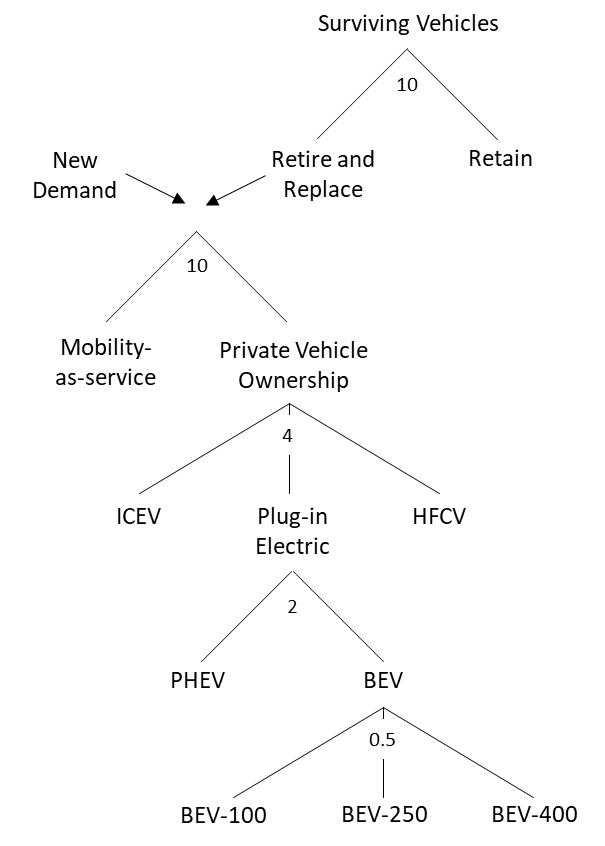
Cost Categories
- Upfront capital costs
- Vehicle purchase cost
- Availability premium
- Policy subsidies
- Charging infrastructure cost
- Home charging equipment and installation
- Public charging investment
- Annual operating costs
- Delivered fuel costs
- Variable maintenance costs (levelized)
- Variable disutility costs (e.g. refueling, range limitation)
- Fixed ownership costs (e.g. insurance and parking)
Vehicle Purchase Costs
New vehicle purchase costs assumptions are based on EPRI estimates and projections of component costs for each individual drivetrain, in particular battery pack and fuel cell system costs. Costs reflect a basic trim level and are primarily intended to represent the relative costs of alternative drivetrain technologies. ICEV costs are assumed to increase slightly over time based on assumed glider efficiency improvements and hybridization. Battery and fuel cell system component costs are assumed to decline significantly over time, falling to roughly 30% of today's levels by 2050 in both cases. The assumed current levels for light-duty vehicle applications are $137/kWh (battery cell cost only) and $181/kW (fuel cell cost only). Note the implication that battery costs scale with energy storage capacity while fuel cell costs scale with power output capacity.
Total vehicle costs for BEV, PHEV, and HFCVs for each light-duty class (car, SUV, pickup truck, and van) are projected based on these assumed component cost declines combined with assumptions about energy and power requirements (with battery size to achieve a given range declining over time based on assumed glider efficiency improvements), vehicle-level economies of scale (e.g ratio between battery cell and pack costs), and a manufacturing cost premium for larger vehicles in initial model time periods to reflect the early stage of the market for alternative vehicles in these segments. HFCV costs also account for a small battery component to manage power delivery to the motor and a compressed hydrogen storage tank. Chart 1 summarizes the vehicle purchase costs for each class and technology.
The reference assumptions for the PHEV and EV range are 40 miles and 250 miles, respectively.
- Chart
- Table
Data Definitions
Measures
- Car ($): Light-duty car cost
- SUV ($): Light-duty sport utility vehicle (SUV) cost
- Truck ($): Light-duty pickup truck cost
- Van ($): Light-duty van cost
Technology
- ICEV: Internal combustion engine vehicle
- PHEV (50 mi.): Plug-in hybrid electric vehicle (50-mile range)
- BEV (100 mi.): Battery electric vehicle (100-mile range)
- BEV (250 mi.): Battery electric vehicle (250-mile range)
- BEV (400 mi.): Battery electric vehicle (400-mile range)
- HFCV: Hydrogen fuel cell vehicle
Availability Premium and Policy Subsidies
To reflect the current limited availability of alternative vehicle technologies in larger vehicle size segments, we assume a temporary availability premium of $10,000 per vehicle in the 2025 and $5,000 in 2030 for the SUV, pickup truck, and van classes. This premium is additional to the vehicle purchase price reflected above in Chart 1.
The model does not currently include explicit subsidies, for example tax credits for alternative vehicle purchases, although these could be incorporated on a scenario basis. Thus the assumed vehicle purchase costs in Chart 1 (plus availability premium) should be interpreted as "after subsidy" costs from the consumer's perspective.
Charging Infrastructure Costs
US-REGEN includes both private and public charging infrastructure costs in the total cost metric for battery electric vehicles. These costs are based on a detailed set of assumptions about both the installed costs of different types of charging stations and the ratio between number of vehicles and number of stations for different structural classes, in particular settlement/building type, charging access category, and vehicle range.
Charging station costs for light-duty vehicles are based on three types of stations:
- Level 2 charging at a single-family home
- $1,000 per station installed cost / $1,500 in urban locations
- Level 2 charging in a public location (either multi-family home garage or commercial location)
- $3,000 per station installed cost / $6,000 in urban locations
- Level 3 DC fast charging in a highway location
- $24,000 per station installed cost
The number of vehicles per station depends on several factors:
- Single-family home with home charging
- 1 vehicle per station for BEVs / 2 for PHEVs
- Multi-family home with on-site public charging
- 1-4 vehicles per station depending on BEV range and access to workplace charging
- Workplace public charging
- 1-4 vehicles per station depending on BEV range and access to home charging
- Destination public charging (Level 2, e.g. at a shopping center)
- 20 vehicles per station for vehicle owners with access to both home and work charging
- 10 vehicles per station for vehicle owners with access to either home or work charging
- 1-2 vehicle per station (depending on BEV range) if solely dependent on public charging
- Highway fast charging
- 30 vehicles per station for all BEVs
Most vehicle owners fall into the category of either home charging only or public charging only, as the current deployment of workplace charging stations is very small. However, workplace charging is growing, and could be an important factor in the diurnal shape of charging load. Additionally, roughly one quarter of vehicles are owned by households living in multi-family or other housing (this share varies by region and is highest in urban locations). Very few of these vehicle owners currently have access to home charging, but the installation of public charging stations located in residential parking structures (effectively home charging at public charging costs for multi-family housing) could increase this share over time. The model assumes that access to workplace charging and access to home charging for multi-family housing both grow over time, as shown in Figure 2.
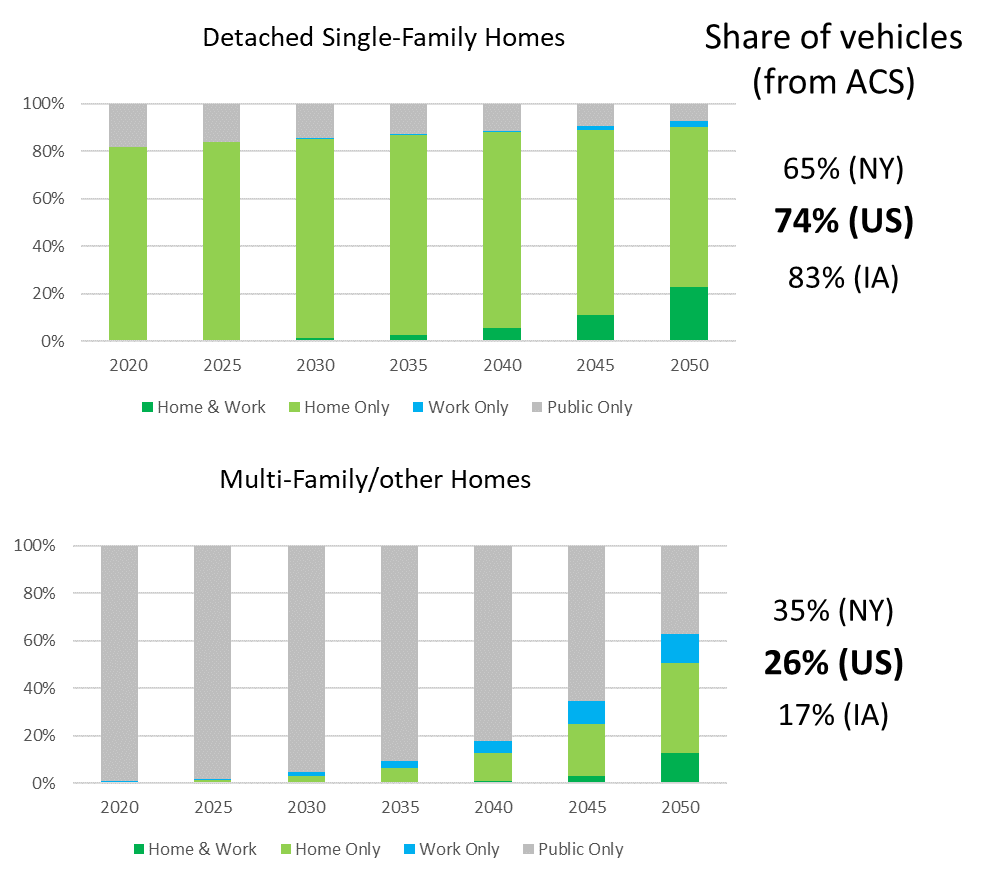
Together, these assumptions translate into an upfront investment cost per vehicle for charging infrastructure based on the characteristics of the vehicle and household making the purchase. There are many possible combinations; four illustrative examples are provided in Table 2.
Home Level 2 | Workplace Level 2 | Public Level 2 | Highway Level 3 | Total | |
---|---|---|---|---|---|
Single-Family Suburban Home Only BEV-250 | 1 @ $1k = $1000 | 0 | 1/10 @ $3k = $300 | 1/30 @ $24k = $800 | $2100 |
Single-Family Urban Work Only BEV-250 | 0 | 1/1.5 @ $6k = $4000 | 1/10 @ $6k = $600 | 1/30 @ $24k = $800 | $5400 |
Multi-Family Urban Home & Work BEV-100 | 1/3 @ $6k = $2000 | 1/3 @ $6k = $2000 | 1/20 @ $6k = $300 | 1/30 @ $24k = $800 | $5100 |
Multi-Family Urban Public Only BEV-400 | 0 | 0 | 1 @ $6k = $6000 | 1/30 @ $24k = $800 | $6800 |
Delivered Fuel Costs
Delivered fuel costs depend on the efficiency of the vehicle, annual vehicle miles traveled, and the delivered price of the fuel. ICEV energy consumption per mile is assumed to fall to roughly 60% of current new vintage technology by 2050 based on both glider efficiency improvements and increased market penetration of non-plug-in hybrid drivetrains (i.e. use of a supplemental internal battery with regenerative braking). Efficiency of BEVs and HFCVs declines more slowly over time to reflect only glider improvements (i.e. hybridization is not applicable), as well as improvements in component-level of efficiencies of batteries and fuel cells. The charging efficiency for BEVs and PHEVs is assumed to increase from 85% with current technology to 94% in 2050, while the fuel cell efficiency in HFCVs is assumed to increase from 53% currently to 64% in 2050. The efficiency of the PHEV in ICEV mode already reflects hybrid operation, thus it declines more slowly than the ICEV technology reflecting the fleet average level of hybridization. Finally, battery electric vehicle efficiency is impacted by the outside temperature, with more energy consumption per mile required at colder temperatures due to both decreased battery performance and increased auxiliary loads for cabin heating. A regional temperature adjustment is applied to both annual fuel costs in the total cost of ownership calculation and to the seasonal profile of charging load. Rated vehicle efficiency assumptions (before the regional temperature adjustment) for each technology type across vintages are shown in Chart 2.
- Chart
- Table
Data Definitions
Measures
- Car (mpg-e): Light-duty car efficiency
- SUV (mpg-e): Light-duty sport utility vehicle (SUV) efficiency
- Truck (mpg-e): Light-duty pickup truck efficiency
- Van (mpg-e): Light-duty van efficiency
Technology
- ICEV: Internal combustion engine vehicle
- PHEV (in ICEV mode): Plug-in hybrid electric vehicle in ICEV mode
- BEV/PHEV (in elec mode): Battery or plug-in hybrid electric vehicle in electric mode
- HFCV: Hydrogen fuel cell vehicle
Vehicle miles traveled (VMT) vary significantly across the vehicle population (based on survey data from NHTS, 2017). Average VMT per vehicle is around 11,000 miles per year, but annual miles per vehicle for primary (i.e. most-used) vehicles in high driving intensity households ranges from 20,000 to 40,000, while tertiary (i.e. least-used) vehicles in low-driving intensity households travel only 1,000 to 2,000 miles per year. Note that the stock turnover model accounts for older vehicles being used less over time, so that new purchases are assumed to be used mainly as primary and secondary vehicles. For PHEVs, the fraction of miles traveled using battery electricity depends on annual vehicle utilization. The assumed battery range for the PHEV technology is 50 miles, which is sufficient to cover nearly all trips for low utilization vehicles, but the electric fraction falls to 50% or lower for higher utilization vehicles. This fraction is estimated assuming a Gamma distribution for daily miles traveled (following the approach in the Market Acceptance of Advanced Automotive Technologies, or MA3T, model from the Oak Ridge National Laboratory).
Delivered prices for electricity are calculated in the electric model on a regional basis. The cost of electricity for home-based charging for single-family homes is based on the residential retail price, while charging in public locations is based on the commercial retail price. The amount of charging in each location depends on charging access category and annual miles traveled. Note that retail prices include levelized T&D costs associated with power delivery. While the cost of the charging infrastructure itself is reflected separately in the model, depending on the public charging business model, these costs may in reality be recovered via electricity sales.
Delivered prices for non-electric fuels are calculated in the fuels model, which represents the upstream production and blending (e.g. of petroleum and bio-fuels) of gasoline and hydrogen. The distribution costs for gasoline are based on the observed mark-up between retail and "refinery gate" prices in historical EIA data and are generally low at around $3/mmbtu. The distribution and dispensing costs for hydrogen are a much more significant component of the delivered price. As discussed in the Fuel Delivery and Storage section, these costs are represented on a levelized basis. The distribution costs for hydrogen are based on pipeline delivery estimated at $8/mmbtu (around $1/kg) for retail re-fueling stations, which is higher than the analogous cost for liquid fuels but much less than the costs of small-scale delivery of compressed or liquefied hydrogen by truck. Additionally, hydrogen re-fueling stations must dispense the fuel into vehicles, which requires installing and maintaining special high-pressure equipment and on-site storage. These costs are estimated to be $24/mmbtu (around $3/kg) on a levelized basis for personal light-duty vehicle hydrogen service stations. While significantly larger than the analogous costs for liquid fuels, this estimate is about half of current hydrogen dispensing costs in California as it reflects learning and economies of scale.
Variable Maintenance Costs
Maintenance is a significant cost component for conventional ICEVs, with average total maintenance costs over the life of a vehicle roughly equal to the initial purchase costs. US-REGEN represents these costs as a linear function of miles traveled, at a rate of $0.20/mile, which is roughly consistent with reported levelized expenditures for existing vehicles. BEVs have much simpler drivetrains with fewer moving parts and are thus expected to incur significantly lower maintenance costs than ICEVs. REGEN assumes a levelized maintenance cost of $0.10/mile for BEVs and $0.15/mile for PHEVs (which include an ICEV drivetrain but use it relatively infrequently). These estimates are consistent with early observations of currently deployed electric vehicles, although more data will become available over time. Similar to BEVs, HFCVs use an electric drivetrain that avoids more costly engine maintenance; REGEN assumes $0.10/mile levelized maintenance for HFCVs as well. For both battery and fuel cell systems, the most significant maintenance expense is the eventual replacement of the battery/fuel cell itself.
Variable Disutility Costs
Trade-offs between alternative vehicle technologies involve complex, multi-attribute decisions. While the REGEN model does not attempt a comprehensive representation of behavioral factors associated with consumer preferences for different vehicle attributes, it does incorporate quantitative assumptions about the disutility costs associated with range limitations and refueling, two salient aspects of the trade-offs between different vehicle technologies. The cost of range limitation applies only to BEVs and depends on annual miles traveled and the range of the BEV. Assuming a Gamma distribution around the daily average, REGEN estimates the number of days the range would be exceeded and assumes $100/day disutility cost (e.g. the cost of renting an alternative vehicle, similar to the approach used in the MA3T model, as described in McCollum, et al 2018).[1] The realized disutility cost values for the 100-mile range option range from less than $100/year for low-utilization vehicles to over $10,000 per year for high-utilization vehicles. For the 250-mile range option, disutility costs fall in the range of $200-$2,000 per year, while the 400-mile range option incurs only $100-$300 per year disutility costs for the highest utilization vehicles. Note that the actual disutility of exceeding a vehicle's range likely depends on the availability of public charging. As noted above, the model also assumes one highway fast charging station per 30 vehicles has been installed (the cost of which is included in the vehicle purchase decision). Thus the additional inclusion of a disutility cost may be over-estimating the economic implications of limited battery range. The primary impact of this cost is to capture the incentives to choose a BEV range option that corresponds to driving intensity, e.g. the 100-mile range is acceptable for low-utilization vehicles, while paying the upfront premium for the 400-mile range is worth it for high-utilization vehicles. Model results suggest that the 250-mile range option is the preferred choice in most cases.
Disutility costs for refueling apply to vehicle types / charging locations that require the owner to make an explicit trip to refuel the vehicle. BEVs and PHEVs that are charged at home or work avoid these costs. For ICEVs, REGEN assumes a disutility cost of $0.01/mile, or approximately $3 per visit to a fueling station. For electric vehicle charging at public locations, REGEN assumes this cost is at $0.02/mile, higher on a levelized basis given the inconvenience of scheduling time to complete the charge. That is, for vehicle owners with access to home or work charging, electric vehicles have a refueling convenience advantage over ICEVs, while those who rely more on public charging have a convenience disadvantage. For HFCVs, REGEN assumes a refueling disutility cost of $0.02/mile to reflect an expected lower density of station availability than today's gasoline service stations.
Fixed Ownership Costs
The non-variable costs of vehicle ownership, such as insurance and parking, are assumed to be equivalent across vehicle types and thus not relevant for trade-offs between vehicles. However, they are relevant for the trade-off between vehicle ownership and the use of a mobility service (to the extent such an option is available). Particularly for low-utilization vehicles, fixed ownership costs can make up a significant share of total ownership costs, enhancing the cost-effectiveness of the mobility-as-service alternative. As shown in Table 4, US-REGEN assumes that fixed costs are higher in urban areas and lowest in rural areas, and increase slightly for higher driving intensity classes based on insurance discounts for lower annual mileage.
Driving Intensity → | Low | Medium | High |
---|---|---|---|
Urban | $1,800 | $2,000 | $2,200 |
Suburban | $1,200 | $1,400 | $1,600 |
Rural | $1,000 | $1,200 | $1,400 |
Mobility-as-a-Service and Autonomous Vehicles
While technical, safety, and regulatory hurdles remain for deployment at scale, the potential economic advantages of mobility-as-a-service options and autonomous vehicles—essentially replacing labor with relatively less expensive capital in the form of sensors and software—are spurring significant investment and strategic shifts throughout the industry. As shown in Figure 1, US-REGEN can represent an autonomous-based mobility service that competes directly with the traditional mode of private vehicle ownership for passenger transport. The economic potential for ridesharing and driverless vehicles derives from higher utilization rates, which offer strong synergies with a low-operating cost electric battery platform. Many low-annual-mileage drivers, particularly in urban environments with high fixed costs of vehicle ownership (such as parking and insurance), would have much lower overall costs using a service than by owning a private vehicle.
In addition to autonomous vehicle services competing against privately owned vehicle purchases, US-REGEN has an autonomous vehicle service model that includes decision variables for the number of vehicles of alternative technology types, including both EVs and ICEVs, number of EV chargers at various speeds, and dispatch of vehicles (and chargers) against an hourly demand profile for vehicle miles traveled. The model is solved as an optimization of vehicle and charging infrastructure capital costs plus fuel and operating costs of vehicle dispatch. The scale of service demand is normalized, as all costs are linear, so that the resulting solution yields an average price per mile for the autonomous vehicle service based on assumptions about costs, fuel prices, and capacity utilization which is a function of the demand profile. This price is used as the input to a vehicle choice model at the household level, in which one option for vehicle purchase is to instead "subscribe" to the autonomous service at the model's calculated price per mile. These components are solved iteratively with a model electric supply, and in scenarios with real-time pricing for the autonomous vehicle fleet, the hourly price is applied as input to the service model's optimization of charging profile.
To account for additional vehicle miles and energy consumption needed to originate passenger trips (known as "deadheading"), the model includes a proportional increase on top of the exogenous assumption for service demand. However, US-REGEN does not include other potential rebound effects, such as increased demand for trips or higher occupancy rates.
Charging Shapes
The passenger vehicle model currently assumes fixed charging shapes for different household types. These profiles are based on the EPRI-NRDC (2015) study "Environmental Assessment of a Full Electric Transportation Portfolio, Volume 2" (EPRI ID 3002006876). As shown in Figure 3, there are three charging profiles, and a mix of these profiles by different household types gives rise to an uncoordinated charging equilibrium. US-REGEN also provides options for flexible charging, including for a fleet of mobility-as-service vehicles.
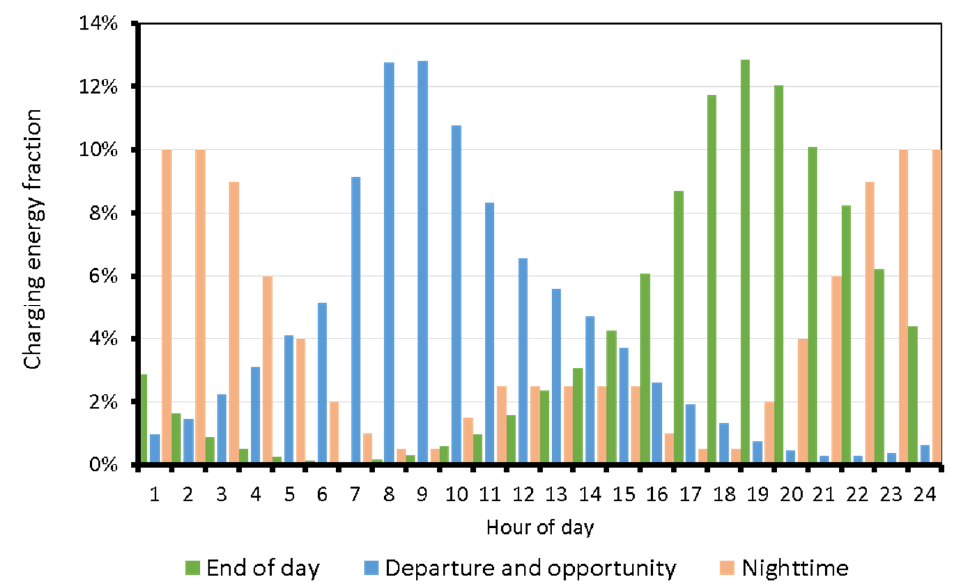
On-Road Fleet and Non-Road Vehicles
US-REGEN represents endogenous adoption of end-use technologies for on-road fleet transportation (primarily medium- and heavy-duty vehicles) and non-road transportation segments based on a cost optimization across alternative vehicle options. The optimization takes into account the stock and vintage structure of the current fleet, projected growth in service demand, technology improvements over time, policy and market impacts on delivered fuel prices (as represented in the corresponding REGEN modules), and policy incentives targeting specific sectors or vehicle technologies. Each segment is modeled separately with a distinct set of vehicle options. Medium- and heavy-duty on-road segments (as well as light-duty fleet vehicles) are organized separately from the non-road segments.
On-Road Fleet Vehicles
US-REGEN breaks down on-road fleet vehicles into the following segments:
- Light duty fleet car (roughly GVWR < 6000)
- Light duty fleet SUV/truck (roughly GVWR bw 6000 and 8500)
- Light duty Class 2 (roughly GVWR bw 8500 and 10000)
- Medium duty Class 3 (GVWR bw 10000 and 14000) Vocational
- Medium duty Class 3 (GVWR bw 10000 and 14000) Local Freight
- Medium duty Class 4-6 (GVWR bw 14000 and 26000) Vocational
- Medium duty Class 4-6 (GVWR bw 14000 and 26000) Local Freight
- Heavy duty Class 7-8 (GVWR > 26000) Vocational
- Heavy duty Class 7-8 (GVWR > 26000) Local Freight (Drayage)
- Heavy duty Class 7-8 (GVWR > 26000) Long-Distance Freight
- School bus
- City/transit bus
- Intercity bus
The candidate technologies for on-road fleet segments include:
- ICEV (including both gasoline and diesel vehicles depending on segment)
- BEV (with an assumed range of 300 miles for most segments, 600 miles for long-distance freight)
- CNGV (expect for light-duty fleet segments)
- HFCV (fuel cell system plus a supplemental battery)
Vehicle Purchase Costs
Similar to the light-duty vehicles model, the upfront capital costs of vehicles in each segment are constructed from component-level assumptions about the vehicle base or glider and each individual drivetrain. Both battery and fuel cell costs are assumed to decline significantly over time. The model also assumes that battery and fuel cell systems for larger vehicle types have an additional cost premium in early time periods that declines over time with experience and scale. The total cost per vehicle assumed in US-REGEN for each on-road fleet segment over time is shown in Chart 3.
- Chart
- Table
Data Definitions
Measures
- Car ($): Light duty fleet car (roughly GVWR < 6000)
- SUV ($): Light duty fleet SUV/truck (roughly GVWR bw 6000 and 8500)
- Class 2 Truck ($): Light duty Class 2 (roughly GVWR bw 8500 and 10000)
- Class 3 (Voc) ($): Medium duty Class 3 (GVWR bw 10000 and 14000) Vocational
- Class 3 (Freight) ($): Medium duty Class 3 (GVWR bw 10000 and 14000) Freight
- Class 3-6 (Voc) ($): Medium duty Class 4-6 (GVWR bw 14000 and 26000) Vocational
- Class 4-6 (Freight) ($): Medium duty Class 4-6 (GVWR bw 14000 and 26000) Freight
- Class 7-8 (Voc) ($): Heavy duty Class 7-8 (GVWR > 26000) Vocational
- Class 7-8 (Freight) ($): Heavy duty Class 7-8 (GVWR > 26000) Freight
- School Bus ($): School bus
- Transit Bus ($): City/transit bus
- Intercity Bus ($): Intercity bus
Technology
- ICEV: Internal combustion engine vehicle
- CNGV: Compressed natural gas vehicle
- BEV: Battery electric vehicle (range depends on class)
- HFCV: Hydrogen fuel cell vehicle
Charging Infrastructure Costs
While the costs of re-fueling infrastructure for non-electric fuels are included in the delivered price of those fuels to the transportation sector, REGEN considers charging infrastructure costs separately from the delivery of electricity. The cost per vehicle for upfront charging infrastructure investment is determined by the assumptions for the assumed cost per station and number of stations per vehicle in each segment. Table 6 summarizes these assumptions for each on-road vehicle category. Cost per station is assumed to decline slightly over time with technological improvements.
Vehicles per Station | Charging Level / kW | Cost per station ($000) | |
---|---|---|---|
Light-duty Car (fleet) | 2 | Level 2 / 12 kW | 2 |
Light-duty SUV/truck (fleet) | 1 | Level 2 / 12 kW | 2 |
Class 2 Truck | 2 | Level 3 DC / 30 kW | 20 |
Class 3 Truck | 2 | Level 3 DC / 60 kW | 40 |
Class 4-6 Truck | 2 | Level 3 DC / 100 kW | 60 |
School Bus | 3 | Level 3 DC / 135 kW | 75 |
Class 7-8 Truck / Bus | 3 | Level 3 DC / 250 kW | 150 |
Annual Operating Costs
Annual operating costs for fuel and maintenance are assumed to scale with vehicle miles traveled. Average annual vehicle miles per vehicle is based on EIA data reported from the NEMS for the base year of recent AEO projections. Available survey data for truck fleet composition and utilization is unfortunately very limited, but older data (e.g. the 2002 VIUS survey) suggests that there is significant variation of vehicle miles per vehicle within fleets and that newer vehicles tend to have higher utilization than older vehicles. To capture these dynamics in a stylized way (i.e. as a placeholder for more detailed data), REGEN assumes that vehicles within each segment are divided into low- and high-driving intensity classes, where vehicle miles per vehicle are 20-50% higher/lower than the fleet average in the respective class. The optimization model can choose to allocate vehicles of different vintages or types to each class so as to minimize total costs.
Fuel costs are determined by vehicle efficiency (i.e. fuel consumption per mile traveled) and the delivered price of fuels. US-REGEN assumptions for vehicle efficiency by segment, vehicle type, and vintage are shown in Chart 4. Delivered prices for electricity are based on electric model outputs, plus a mark-up for T&D costs consistent with observed commercial retail prices. In future model versions, this cost could be determined based on a more detailed assessment of the implied load factor for fleet charging vs other commercial loads. As discussed in the light-duty vehicle model section, the delivered costs of non-electric fuels are based on the upstream cost as assessed in the fuels model plus a levelized distribution cost. For liquid fuels the distribution cost is based on observed retail price mark-ups for gasoline and diesel. The distribution costs for hydrogen are based on pipeline delivery estimated at $8/mmbtu (around $1/kg) for fleet re-fueling stations, plus dispensing costs of $16/mmbtu (around $2/kg) on a levelized basis (lower than for personal light-duty vehicles based on larger scale for fleet vehicles).
- Chart
- Table
Data Definitions
Measures
- Car (mpg-e): Light duty fleet car (roughly GVWR < 6000)
- SUV (mpg-e): Light duty fleet SUV/truck (roughly GVWR bw 6000 and 8500)
- Class 2 Truck (mpg-e): Light duty Class 2 (roughly GVWR bw 8500 and 10000)
- Class 3 (Voc) (mpg-e): Medium duty Class 3 (GVWR bw 10000 and 14000) Vocational
- Class 3 (Freight) (mpg-e): Medium duty Class 3 (GVWR bw 10000 and 14000) Freight
- Class 3-6 (Voc) (mpg-e): Medium duty Class 4-6 (GVWR bw 14000 and 26000) Vocational
- Class 4-6 (Freight) (mpg-e): Medium duty Class 4-6 (GVWR bw 14000 and 26000) Freight
- Class 7-8 (Voc) (mpg-e): Heavy duty Class 7-8 (GVWR > 26000) Vocational
- Class 7-8 (Freight) (mpg-e): Heavy duty Class 7-8 (GVWR > 26000) Freight
- School Bus (mpg-e): School bus
- Transit Bus (mpg-e): City/transit bus
- Intercity Bus (mpg-e): Intercity bus
Technology
- ICEV (mgs): Internal combustion engine vehicle (motor gasoline)
- ICEV (dsl): Internal combustion engine vehicle (diesel)
- CNGV: Compressed natural gas vehicle
- BEV: Battery electric vehicle
- HFCV: Hydrogen fuel cell vehicle
Non-fuel operating and maintenance costs are assumed to be $0.15/mile for ICEV vehicles in most segments, with a higher cost of $0.25/mile assumed for vocational trucks and transit busses to account for more intensive duty cycles and non-driving fuel use. Similar to the light-duty vehicle model, BEVs are assumed to have maintenance costs of half the ICEV level, while HFCV maintenance costs are assumed to be 70% of the ICEV level and CNGV costs are equivalent to ICEV costs.
Non-Road Vehicles
US-REGEN breaks down non-road transportation into the following segments:
- Short-haul aviation (<600 miles)
- Long-haul domestic aviation
- International aviation
- Military aviation
- Domestic maritime shipping
- Passenger maritime
- Recreational maritime
- International shipping (bunker fuels)
- Freight rail
- Passenger intercity rail
- Commuter rail
- Transit rail
- Non-road vehicles in agriculture
- Non-road equipment in agriculture
- Non-road vehicles in construction
- Non-road equipment in construction
- Non-road vehicles in non-energy mining
- Non-road equipment in non-energy mining
- Non-road vehicles and equipment for military (excluding jet fuel)
The candidate technologies for non-road vary by segment but include the following:
- Conventional (including gasoline, diesel, and jet fuel vehicles depending on segment)
- Electric (all except long-haul aviation, shipping, and freight and passenger rail)
- CNG/LNG (shipping and passenger maritime, freight rail, and some non-road equipment)
- Hydrogen (all except long-haul aviation)
- Ammonia (shipping and maritime and some non-road vehicles)
Each technology is characterized in terms of capital costs, energy consumption, and non-energy operating cost per service unit. Additionally, electric technologies include costs for charging equipment. Costs and efficiency can improve over time, and some technologies are assumed to be unavailable until future years. US-REGEN assumptions for these parameters are based on reports from the literature, where possible, or adapted from assumptions in similar sectors. In general there is significant uncertainty and need for additional research around the potential cost and performance of alternative vehicle and equipment technologies in the non-road segments
Aviation Technology Cost and Performance Assumptions
Only short-haul aviation (trips less than 600 miles) is assumed to have the possibility of using battery electric or hydrogen-powered aircraft. Domestic long-haul, international, and military aviation is assumed to use only jet fuel (which could be produced from petroleum, bioenergy, or hydrogen and carbon synthesis). Based on airline trip data, the share of current service demand (in terms of airline seat miles) and associated fuel use attributed to short-haul trips is around 10%, and AEO projections of air travel service demand suggest that this share will fall over time. Thus the potential for substitution of electricity and hydrogen for jet fuel is limited in the aviation sector.
US-REGEN assumes that electric and hydrogen-powered aircraft would not be available until 2035, at which point the cost of an electric aircraft would be roughly equivalent to the cost of a comparable (short-haul) conventional aircraft, while a hydrogen-powered aircraft would be roughly 15% more expensive. However, the charging equipment needed for the electric aircraft would have a capital cost of roughly 20% of the cost of the aircraft. Similar to on-road vehicles, an electric aircraft would be significantly more efficient in terms of final energy per service unit than a conventional aircraft, while a hydrogen aircraft would fall in between. These assumptions are summarized in Figure 4.
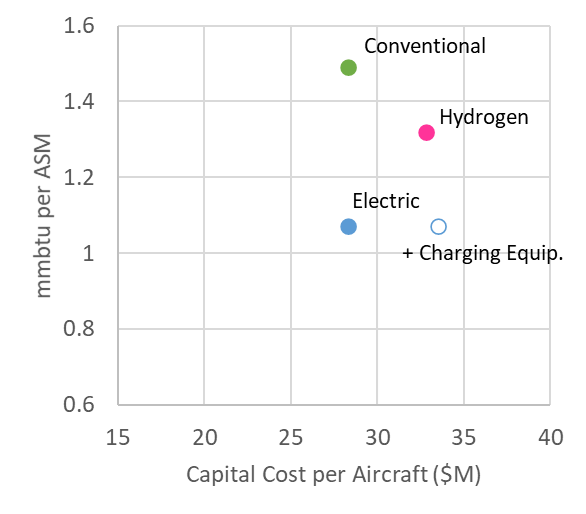
US-REGEN also assumes that efficiency improvements in aircraft materials and design will lower fuel use per seat-mile for all aviation technologies. By 2050, the energy consumption per seat-mile of new vintage aircraft is projected to be roughly half of the current fleet average. Thus, despite a near-doubling of service demand, projected total use of jet fuel slightly declines, even without any potential substitution to electricity or hydrogen for the short-haul segment.
Maritime Technology Cost and Performance Assumptions
US-REGEN assumptions for the capital cost of alternative technologies in the maritime segments, expressed relative to the incumbent petroleum-based technology, are shown in Table 8 for both 2035 and 2050. The relative costs of alternative technologies are assumed to decline over time. Blank cells in the table indicate ineligible technologies for that segment. Table 9 shows assumptions for the relative efficiency of alternative technologies, which do not change over time in relative terms. However, efficiency improvements across all technologies are assumed at a rate of 0.5% per year for recreational, 1% per year for domestic freight and passenger maritime, and 1.5% per year for international shipping.
Diesel | Gasoline | LNG/CNG | Electric | Hydrogen | Ammonia | |
---|---|---|---|---|---|---|
Recreational | 1 | 1.88 | 1.39 | 1.47 | 1.27 | 1.15 | 1.15 | ||
Passenger | 1 | 0.8 | 0.8 | 1.88 | 1.39 | 1.47 | 1.27 | 1.15 | 1.15 | |
Domestic Freight | 1 | 1.05 | 1.05 | 2.58 | 1.90 | 1.43 | 1.23 | ||
International | 1 | 1.07 | 1.07 | 3.62 | 2.67 | 1.38 | 1.19 |
Diesel | Gasoline | LNG/CNG | Electric | Hydrogen | Ammonia | |
---|---|---|---|---|---|---|
All Classes | 1 | 1 | 1.36 | 0.33 | 1 | 1 |
Rail Technology Cost and Performance Assumptions
US-REGEN assumptions for the capital cost of alternative technologies in the rail segments, expressed relative to the incumbent petroleum-based technology, are shown in Table 10 for both 2035 and 2050. The relative costs of alternative technologies are assumed to decline over time. Blank cells in the table indicate ineligible technologies for that segment. Note that transit rail (e.g. urban subway systems) is not shown as it is assumed to be all-electric. Table 11 shows assumptions for the relative efficiency of alternative technologies, which generally do not change over time in relative terms, except that hydrogen rail technologies. Efficiency improvements for diesel and electric technologies are assumed to occur at a rate of 0.5% per year, while hydrogen technologies improve at a rate of 1% per year.
Diesel | Diesel Hybrid | CNG | Electric | Hydrogen | |
---|---|---|---|---|---|
Commuter | 1 | 1.06 | 1.06 | 1.02 | 1.00 | 1.01 | 1.01 | 1.10 | 0.96 |
Passenger | 1 | 1.06 | 1.06 | 1.03 | 1.02 | 1.15 | 1.01 | |
Freight | 1 | 1.57 | 1.55 | 1.91 | 1.43 |
Diesel | Diesel Hybrid | CNG | Electric | Hydrogen | |
---|---|---|---|---|---|
All classes | 1 | 0.8 | 1 | 0.24 | 0.65 | 0.6 |
Other Non-road Vehicles and Equipment
There are several non-road segments that can be difficult or ambiguous to categorize and thus are less well understood and represented in energy system models. Nonetheless these segments constitute significant fuel demands and emissions sources. These segments include non-road vehicles and equipment used in non-manufacturing industries, namely agriculture and forestry, construction, and mining (here we refer specifically to non-energy mining, i.e. metals and minerals, as energy used in the upstream energy sectors is represented in the fuels model). These industries employ many types of vehicles and equipment for a diverse array of end-uses, which is challenging to represent in a model with a detailed structure. US-REGEN divides these activities into two aggregate classes corresponding to vehicles (calibrated to current diesel use) and equipment (calibrated to current use of other fuels), with some specific examples shown in Table 12.
Vehicles (current diesel use) | Equipment (current other fuel use) | |
---|---|---|
Agriculture | Tractors, combines | Tillers, sprayers, grain dryers, space heaters, irrigation pumps |
Construction | Loaders, dozers, excavators | Tampers, plate compactors, paving equipment, saws |
Mining (metals and minerals, excludes coal, oil, and gas extraction) | Haul trucks | Dewatering, ventilation, beneficiation and processing |
US-REGEN assumptions for the capital cost of alternative technologies in the agriculture, construction, and mining non-road segments, expressed relative to the incumbent fossil-based technology, are shown in Table 13. The relative costs of alternative technologies are assumed to decline relative to today but achieve target levels by 2035. The model considers battery electric and hydrogen fuel-cell technologies for all segments, and an ammonia technology for non-road vehicles. Table 14 shows assumptions for the relative efficiency of alternative technologies, which do not change over time in relative terms. However, efficiency improvements across all technologies are assumed at a rate of 1% per year.
Fossil | Electric | Hydrogen | Ammonia | ||
---|---|---|---|---|---|
Non-Road Vehicles | Agriculture | 1 | 1 | 1.1 | 1.2 |
Construction | 1 | 1.1 | 1.2 | 1.3 | |
Mining | 1 | 1.1 | 1.2 | 1.3 | |
Non-Road Equipment | Agriculture | 1 | 1 | 1.2 | |
Construction | 1 | 1 | 1.2 | ||
Mining | 1 | 1.2 | 1.2 |
Fossil | Electric | Hydrogen | Ammonia | |
---|---|---|---|---|
Non-Road Vehicles (all classes) | 1 | 0.3 | 0.5 | 1 |
Non-Road Euipment (all classes) | 1 | 0.3 | 1 |
Mobile equipment in agriculture, construction, and industry presents unique challenges for electrification. In addition to the capital cost of the vehicle or equipment, battery electric technologies incur costs for charging infrastructure, which REGEN assumes are approximately 7 cents per kWh, or roughly twice the levelized cost of charging equipment for on-road vehicles to account for irregular usage and distance from charging base. REGEN also assumes higher operating costs to account for time needed to charge and battery weight, which depending on the duty cycle of the equipment can have a large impact on operations. However, these costs are partially offset by lower maintenance costs for electric vehicles, so that total non-energy operating costs. Hydrogen fuel-cell vehicles do not incur additional costs for charging or operations, although the delivered fuel price reflects costs for dispensing fuel to vehicles at pressure. Maintenance of hydrogen non-road vehicles is assumed to be similar to conventional ICEVs.
McCollum, D. et al (2018). Interaction of consumer preferences and climate policies in the global transition to low-carbon vehicles, Nature Energy 3, 664–673. ↩︎